Mechanical Ventilation in Ventilator
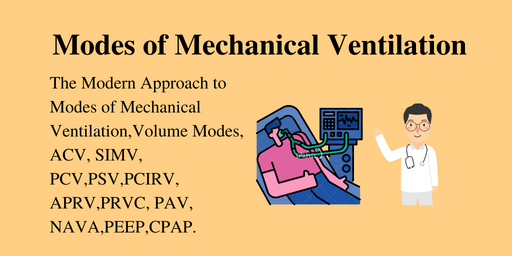
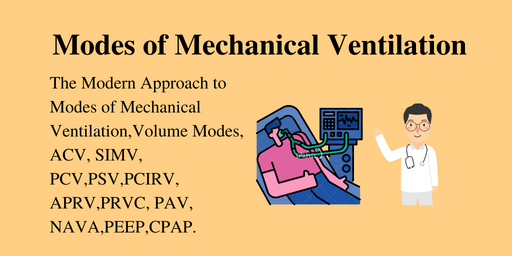
The Modern Approach to Modes of Mechanical Ventilation
While modes have classically been divided up into pressure or volume controlled modes, a more modern approach describes ventilatory modes based on three characteristics the trigger (flow versus pressure), the limit (what determines the size of the breath), and the cycle ( which actually ends the breath).
In both VCV and PCV,
time is the cycle, the difference being in how the time to cessation is
determined. PSV, by contrast, has a flow cycle.
Note also that the lines between
pressure and volume controlled methods are being continuously blurred by
increasingly complex modes. If alarms and backup modes are properly set, the
“disadvantages” of classic modes ( eg possibility of insufficient minute
ventilation in PCV) can be essentially eliminated
For historical reasons, the
following modes will be separated into volume controlled, pressure controlled,
and other modes
Volume Modes
Assist-Control Ventilation (ACV)
Also known as continuous mandatory ventilation (CMV). Each breath is either an assist or control breath, but they are all of the same volume. The larger the volume, the more expiratory time required. If the I:E ratio is less than 1:2, progressive hyperinflation may result.
ACV is particularly undesirable for patients who breathe rapidly they may induce both hyperinflation and respiratory alkalosis. Note that
mechanical ventilation does not eliminate the work of breathing, because the
diaphragm may still be very active.
Synchronized Intermittent-Mandatory Ventilation (SIMV)
Guarantees a certain number of breaths, but unlike ACV, patient breaths are partially their own, reducing the risk of hyperinflation or alkalosis. Mandatory breaths are synchronized to coincide with spontaneous breaths.
Disadvantages of SIMV are increased work of breathing
and a tendency to reduce cardiac output, which may prolong ventilator
dependency. The addition of pressure support on top of spontaneous breaths can
reduce some of the work of breathing. SIMV has been shown to decrease cardiac
output in patients with left-ventricular dysfunction [Crit Care Med 10: 423,
1982]
Stroke vs. SIMV
Personal preference prevails,
except in the following scenarios: 1. Patients who breathe rapidly on ACV
should switch to SIMV 2. Patients who have respiratory muscle weakness and/or
left-ventricular dysfunction should be switched to ACV
Pressure Modes
Pressure-Controlled Ventilation
(PCV)
Less risk of barotrauma as compared to ACV and SIMV. Does not allow for patient-initiated breaths. The inspiratory flow pattern decreases exponentially, reducing peak pressures and improving gas exchange [Chest 122: 2096, 2002].
The biggest disadvantage is
that there are no guarantees for volume, especially when lung mechanics are
changing. Thus, PCV has traditionally been preferred for patients with
neuromuscular disease but otherwise normal lungs.
Pressure Support Ventilation (PSV)
Allows the patient to determine
inflation volume and respiratory frequency (but not pressure, as this is
pressure-controlled), thus can only be used to augment spontaneous breathing.
Pressure support can be used to overcome the resistance of ventilator tubing in
another cycle (5 – 10 cm H20 are generally used, especially during weaning), or
to increase spontaneous breathing. PSV can be delivered through specialized
face masks.
Pressure Controlled Inverse Ratio Ventilation (PCIRV)
Pressure controlled ventilatory
mode in which the majority of time is spent at the higher (inspiratory)
pressure. Early trials were promising, however the risks of auto PEEP and
hemodynamic deterioration due to the decreased expiratory time and increased
mean airway pressure generally outweight the small potential for improved
oxygenation
Airway Pressure Release Ventilation (APRV)
Airway pressure release ventilation is similar to PCIRV – instead of being a variation of PCV in which the I:E ratio is reversed, APRV is a variation of CPAP that releases pressure temporarily on exhalation. This unique mode of ventilation results in higher average airway pressures.
Patients are able to spontaneously ventilate at both
low and high pressures, although typically most (or all) ventilation occurs at
the high pressure. In the absence of attempted breaths, APRV and PCIRV are
identical. As in PCIRV, hemodynamic compromise is a concern in APRV.
Additionally, APRV typically requires increased sedation
Dual Modes
Pressure Regulated Volume Control
(PRVC)
A volume target backup is added
to a pressure assist-control mode
Interactive Modes
Proportional Assist Ventilation
(PAV)
During PAV, the clinician sets
the percentage of work of breathing to be provided by the ventilator. PAV
uses a positive feedback loop to accomplish this, which requires
knowledge of resistance and elastance to properly attenuate the signal
Compliance and resistance must
therefore be periodically calculated – this is accomplished by using intermittent
end-inspiratory and end-expiratory pause maneuvers (which also calculate
auto PEEP). In addition to percent support, the clinician sets the trigger and
the cycle (what actually ends the breath)
The theoretical advantage of PAV
is increased synchrony compared to PSV (which provides the same amount of
support regardless of how much effort the patient makes)
Proportional Assist Ventilation:
Summary
·
Independent Variables : % WOB; trigger; cycle
·
How It Works : positive feedback loop
(requires calculation of resistance and elastance)
·
Theoretical Advantage(s) : better synchrony
Neurally Adjusted Ventilatory
Assist (NAVA)
Additional Modes, Strategies,
Parameters
Inverse Ratio Ventilation
Inverse Ratio Ventilation (IRV) is a subset of PCV in which inflation time is prolonged (In IRV, 1:1, 2:1, or 3:1 may be used. Normal I:E is 1:3). This lowers peak airway pressures but increases mean airway pressures.
The result may be improved oxygenation but at
the expense of compromised venous return and cardiac output, thus it is not
clear that this mode of ventilation leads to improved survival. IRV's major
indication is in patients with ARDS with refractory hypoxemia or hypercapnia in
other modes of ventilation [Am J Surg 183: 151, 2002]
Adaptive Support Ventilation
Calculates the expiratory time
constant in order to guarantee sufficient expiratory time and thus minimize air
trapping
Tube Compensation
Positive End Expiratory Pressure
(PEEP)
Note: PEEP is not a ventilatory
mode in and of itself
Does not allow alveolar pressure to equilibrate with the atmosphere. PEEP displaces the entire pressure waveform, thus mean intrathoracic pressure increases and the effects on cardiac output are amplified. Low levels of PEEP can be very dangerous, even 5 cm H20, especially in patients with hypovolemia or cardiac dysfunction.
When measuring the effectiveness of PEEP, cardiac output must always be calculated because at high saturations, changes in Q will be more important than SaO2 never use SaO2 as an endpoint for PEEP. The effects of PEEP are not caused by the PEEP itself but by its effects on Ppeak and Pmean , both of which it increases.
Risk
of barotrauma is dependent on Ppeak , while cardiac output response depends on
Pmean . In fact, in a recent study of ARDS patients, it was shown that
increasing PEEP from 0 to 5, 10, and 15 cm H2O was met with corresponding
decreases in CO [Crit Care Med 31: 2719, 2003]
PEEP is clinically indicated for 1) low-volume ventilation cycles 2) FiO2 requirements > 0.60, especially in stiff, diffusely injured lungs such as ARDS and 3) obstructive lung disease.
Do
NOT use in pneumonia, which is not diffuse, and where PEEP will adversely
affect healthy tissue and worsen oxygenation. One way to gauge the effect of
PEEP is to look at peak inspiratory pressure (PIP) – if PIP increases less than
the added PEEP, then the PEEP improved the compliance of the lungs.
A recent phenomenon in the understanding of PEEP is the principle of recruitable lung volume: while this cannot be calculated, it can be estimated by looking at CT scans: atelectasis containing air is recruitable, that devoid of air is not, the idea being only apply PEEP to recruitable lungs, otherwise you may just be inducing ARDS [NEJM 354: 1775, 2006].
The effects of PEEP can also be monitored by tracking the
PaO2/FiO2 ratio (it should increase).
ARDSnet II: 8.3 vs. 13.2 cm H2O:
in patients with acute lung injury and ARDS who receive mechanical ventilation
with a tidal-volume goal of 6 ml per kilogram of predicted body weight and an
end-inspiratory plateau-pressure limit of 30 cm of water, clinical outcomes are
similar whether lower or higher PEEP levels are used [NEJM 351: 327, 2004]
PEEP should not be used
routinely. It does not reduce lung edema (can cause it) or prevent mediastinal
bleeding.
Continuous Positive Airway Pressure (CPAP)
Positive pressure given
throughout the cycle. It can be delivered through a mask and is can be used in
obstructive sleep apnea (esp. with a nasal mask), to postpone intubation, or to
treat acute exacerbations of COPD
Prone Ventilation
May improve oxygenation by redistributing pulmonary blood flow, however a multicenter, randomized trial of 304 patients showed that this improved oxygenation is not accompanied by a change in survival [NEJM 345: 568, 2001] – this was corroborated by two smaller, subsequent randomized controlled trials, which showed an insignificant trend towards improved mortality [J Trauma 59: 333, 2005; Am J Respir Crit Care Med 173: 1233, 2006].
This may not hold for neurosurgery patients – in a study
of 16 SAH (H&H 3 or higher) patients in ARDS, PaO2 increased from 97.3 to
126.6 mm Hg in the prone position and brain tissue oxygen partial pressure
increased from 26.8 to 31.6 mm Hg ( both p <.0001), despite the fact that ICP
increased from 9.3 to 14.8 mm Hg and CPP decreased from 73.0 to 67.7 (both p
<.0001) [Crit Care Med 31: 1831, 2003]
High Frequency Oscillatory Ventilation
In one study of 5 patients with
TBI and ARDS (390 datasets of ICP, CPP, PaCO2 collected), treated HFOV with –
ICP increased in 11 of 390 datasets, CPP was reduced (<70 mmHg) in 66 of
390, and P( a) CO2 variations (<4.7 kPa; >6.0 kPa) were observed in 8.
All these alterations were responsive to treatment. PaO2/FIO2 improved in four
patients [Acta Anaes Scand 49:209, 2005]
High Frequency Percussive Ventilation
10 severe TBI patients with a
Glasgow Coma Score (GCS) < 9, placed on HFPV. There was an increase in PF
ratio (91.8 to 269.7, p < 0.01), PEEP (14 to 16 +/- 3.5), and mean airway
pressure (20.4 to 23.6) 16 hours after institution of HFPV. There was a
decrease in ICP (30.9 to 17.4, p < 0.01), PC02 (37.7 to 32.7, p < 0.05),
and PIP (49.4 to 41, p < 0.05) at 16 hours [J Trauma 57: 542, 2004]
Give your opinion if have any.